The reverberation time in a room is an important criteria for its sound. Generally speaking, the larger the room and the more reflective its surfaces, the longer it takes for the reverberation to decay.
Too much reverberation can lead to bad voice intelligibility. Music can sound blurry and washed-out, and details get lost in the reverb tail. On the other hand, a room with a very short reverberation time can sound dull and lifeless.
Reverberation is created when sound waves are reflected by walls, ceilings, floors, furniture and other objects. Ultimately, the sonic energy is converted to heat energy, and the air itself also contributes to dampening.
In studio control rooms, a reverberation time of about 0.15 to 0.3 seconds is considered ideal. In recording rooms, the reverberation time rarely exceeds 0.5 seconds. Opera houses and concert halls often have reverberation times of 1.5 to 2 seconds. The Cologne Cathedral has an average reverberation time of 12 seconds!
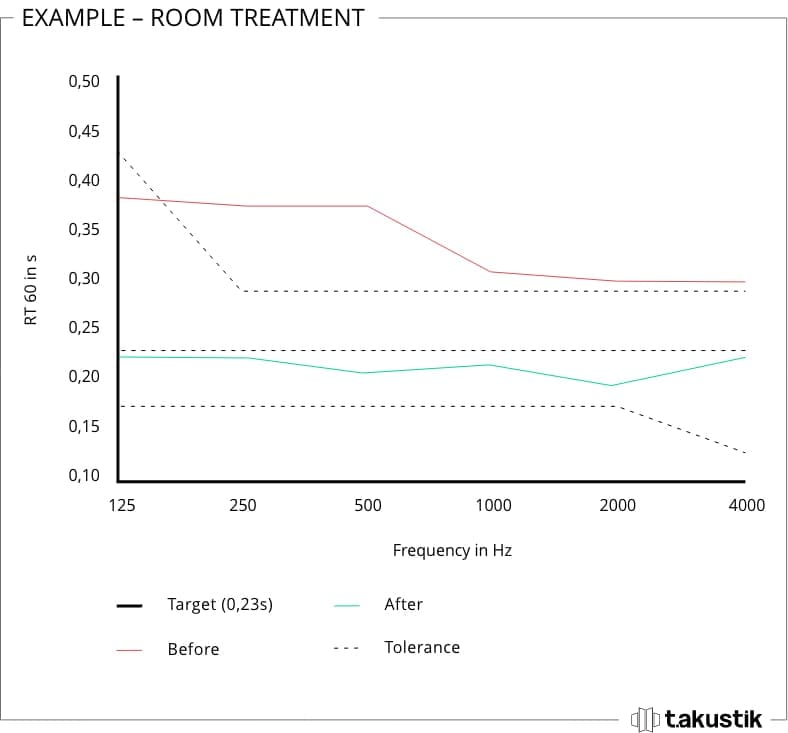
In most rooms, it’s possible to measure the reverberation time. This requires a short impulse like a gunshot or even a hand clap. The reverberation time is the time it takes for the initial sound pressure to decay to one thousandth. This corresponds to a reduction by 60 dB, which is why the reverberation time determined by this method is called “RT60” (Release Time / 60 dB).
In many larger rooms, the RT60 time is too long. In order to reduce the reverberation time, it’s possible to remove sonic energy by installing absorbers. In most studio rooms, however, reverberation is a much smaller problem in practice than early reflections and room modes.
Why are acoustics so important in the office?
Some office work is carried out in single-person offices or at home. However, many workplaces are located in group or open-plan offices. While this facilitates communication between employees, it can also become a nuisance. Noise is the most frequently mentioned disruptive factor among office workers. Excessive noise levels can adversely affect concentration, efficiency, and health.
What may seem like a contradiction, can add up to a positive effect: Acoustic treatment in the office can help to create a balance between effortless communication and undisturbed, stress-free, and efficient working conditions. Nobody is interested in employees who don’t feel good.
While acoustics is a complex field, some terms and relationships that apply to office acoustics are easy to understand.
Reverberation Time
The reverberation time is the most important parameter for measuring the acoustic quality of a room. If the reverberation time is too long, the noise level increases and the voice intelligibility decreases. If it is too short, the voice intelligibility increases throughout the entire room. A very short reverberation time is ideal for conference rooms, but less desirable for open-plan offices because it means that conversations at other desks will be heard clearly.
Lombard-Effect
While the term “Lombard Effect” is not as well-known, everybody has experienced it. When the level of background noise is high, people tend to speak louder, in order to be understood clearly. If several people are talking at the same time, this can build up and result in an even higher noise level. When this occurs continuously in an office over the course of the whole workday, that’s a huge problem.
Cocktail Party Effect
The cocktail party effect describes the ability of the human hearing to focus on a single sound source when several sound sources are present. Background noise and irrelevant acoustic information are suppressed. While it is effective, it means that the brain has to work hard – and continuous stress has an adverse effect on efficiency.
Comb filtering can be heard and measured with spectral meters. The frequency curve has “dips” at regular intervals, which look like a comb. That’s the definition of a comb filter effect. It should be avoided, especially in the listening position, because it can severely color the signal and give it an indirect or “phased” sound.
The comb filter effect occurs when an audio signal is mixed with a delayed signal. The resulting interferences cause cancellation and summing effects. The “detour” that the delayed signal takes determines the distribution of the cancellations and peaks in the frequency band. The lower the level of the delayed signal and the more it’s changed compared to the original, the less comb filtering is introduced.
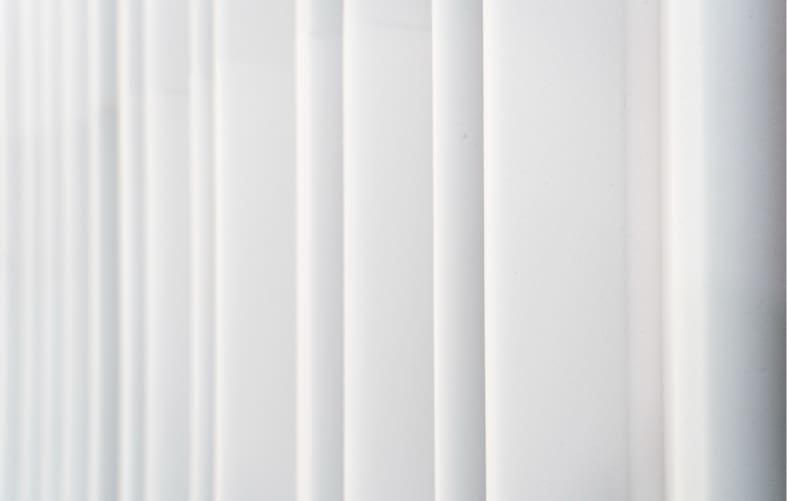
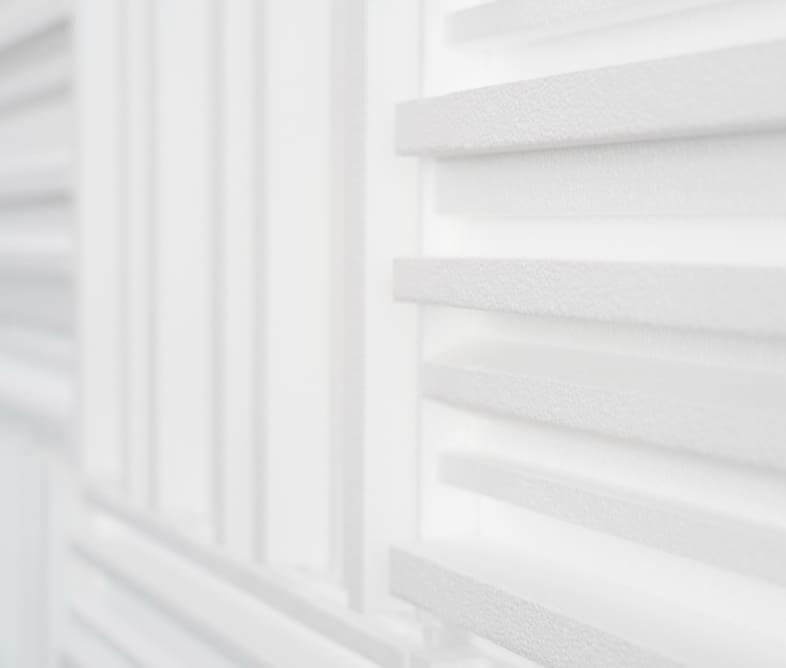
One of the most recognizable examples of the comb filter effect is the reflection that meets the direct signal from the speakers in the ear. Part of the signal reaches the ear directly, while another part takes a detour via the surface of the desk or mixing console. To avoid this, it can help to place the speakers on stands, or even just place them at an angle.
Comb filter effects caused by hard reflections of the speaker signal on side walls, the wall behind the speakers or the ceiling. This type of comb filter effect should be avoided.
One way to achieve this is to use absorbers to prevent these reflections from occurring. Absorbers absorb sonic energy in a wide frequency band. Another option is to diffuse the signal instead of absorbing it. Rather than reducing sonic energy, diffusors divert it into many directions.

The frequencies that we can hear can be divided into several frequency bands. Everyone has heard the terms bass, mids and treble (highs). Sound consists of oscillating waves that propagate through the air. The frequency at which a wave oscillates is measured in Hertz (1 Hz = one oscillation per second). The higher the frequency, the higher the pitch. 20 Hz is in the very low sub bass range and can only be produced by very few instruments. Above 10 kHz, there are no fundamentals; it’s all harmonics and noise components.
It’s important to know that our perception of pitch is not linear, but logarithmic. 200 Hz sounds twice as high as 100 Hz, but 2000 Hz also “only” sounds twice as high as 1000 Hz, even though the difference is 1000 Hz instead of 100 Hz. This is why the bass or low frequency range ranges from 20 Hz to about 200 Hz, the mid range contains frequencies from 200 Hz to 2 kHz and the range from 2 kHz all the way to 20 kHz is the high frequency range.
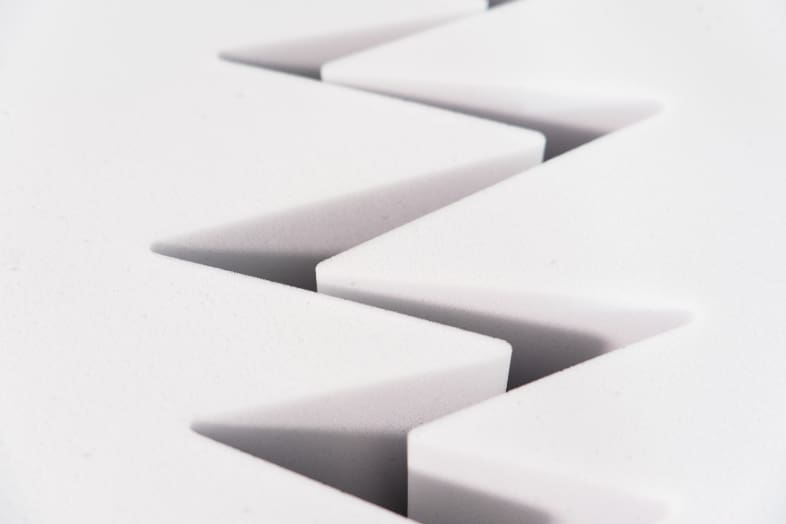
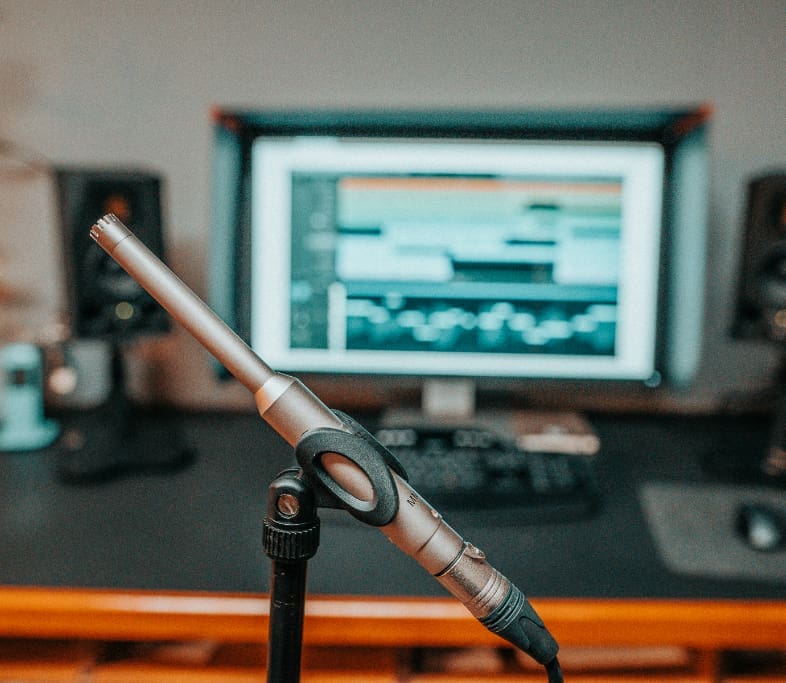
In room acoustics, the low frequency range is treated differently from the mid and high frequency ranges. This isn’t due to fundamentally different physical properties. But in the low frequency range, the wave lengths are so long that it’s not practical to pretend that sound behaves like light, which works in the high frequency range. Some low frequencies can be hard to dampen and can linger in the room for a long time.
These modes aren’t necessarily a bad thing per se. But they become a problem when they cause a non-linear reverberation in the low frequency range, where certain frequencies stand out. This applies especially to situations where modes accumulate at some points in the frequency spectrum, while holes appear elsewhere.
To further complicate things, rooms don’t behave homogeneously in the low frequency range. There may be local build-ups or attenuations. Generally speaking, the low frequencies are most pronounced near the walls, ceiling, floor and in the corners. In order to achieve a uniform reverberation, it’s possible to target the low frequencies with special absorbers (bass traps).
For the most part, the speakers used in music production are different from the ones used for pleasure listening. Studio monitors have certain characteristics that differ from their hi-fi counterparts. First and foremost, nearly all modern studio monitors are active (powered) speakers with built-in amplifiers. Most hi-fi speakers are passive and must be connected to an external amplifier.
The requirements for studio monitors are different from hi-fi speakers. In the studio, a linear response and wide frequency range are essential for assessing problems in recordings and mixes. They’re meant for listening work rather than enjoyment. Conversely, the speakers used for hi-fi listening are mainly supposed to “sound good”. Many hi-fi speakers deliberately exaggerate the low frequency range, but fail to accurately reproduce the very low sub bass range. Others have a strong treble range but neglect the “airy” frequencies just below 20 kHz.
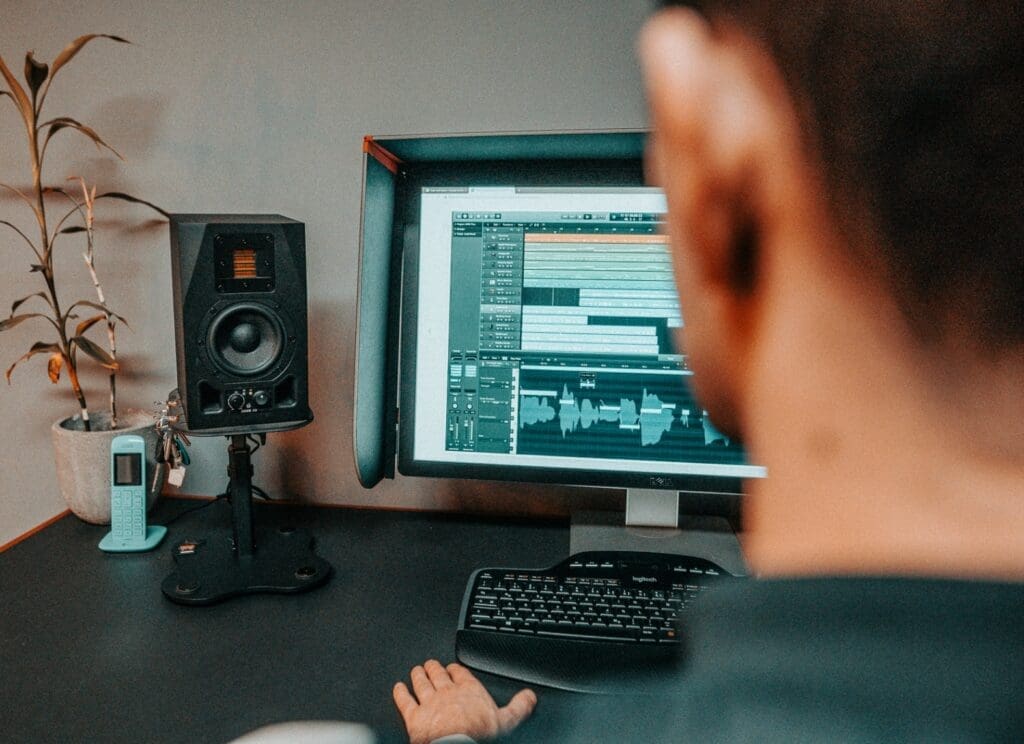
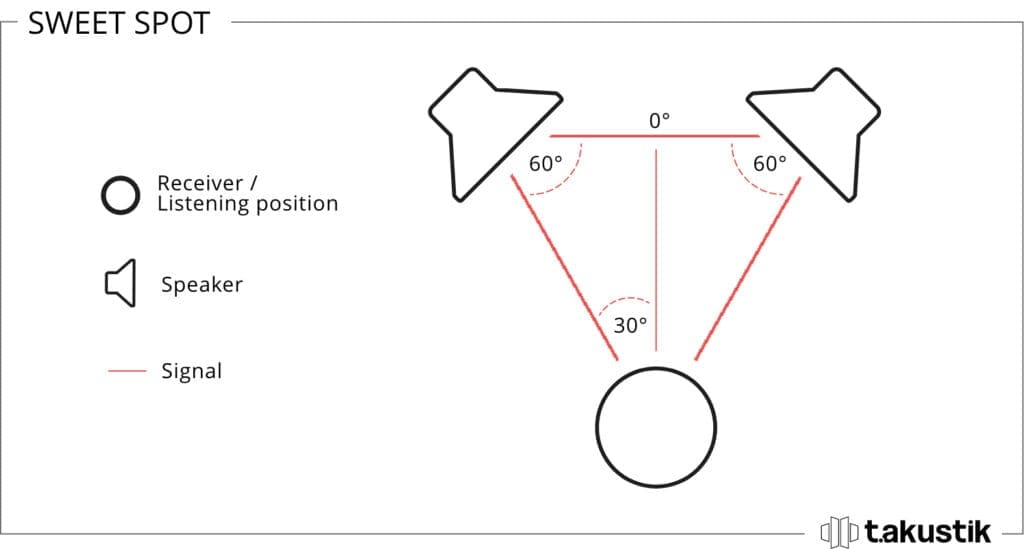
This being said, a completely linear response and accurate impulse reproduction can cause listening fatigue during long sessions. This is why the “sharpness” band is slightly rolled of even in many studio monitors.
The placement and orientation of studio monitors depends on the circumstances and the room. But there are a few simple rules. Firstly, follow the recommendations of the manufacturer to adjust the speaker’s settings. For example, speakers might feature filters for use on a desktop or close to a wall. The most common way to set up studio monitors is the so-called stereo triangle. In this arrangement, the two loudspeakers and the listening position form an isosceles (not just equiangular!) triangle.
It is important to choose monitors that suit the room and speaker arrangement. Near field monitors should not be placed too far from the listening position. Conversely, mid field and far field monitors are optimized for longer distances and placing them too close to the listening position doesn’t make much sense. Loudspeakers are among the most important tools in the studio, so they’re not something you’d want to compromise on.
Furthermore, it always takes a while to get used to new speakers and get to know them. Frequently changing or upgrading monitors isn’t ideal – it’s much better to make a single investment in high-quality speakers! Monitors should offer high power reserves and a wide frequency response. Other criteria are impulse fidelity, stereo imaging, a tight bass response and high detail.
But without good room acoustics, even the best studio monitor cannot live up to its full potential. Uncontrolled early reflections can cause comb filter effects, which can severely impair the listening experience. Avoid this by installing one of our sets for the acoustic treatment of your room: Set finder for studio rooms.
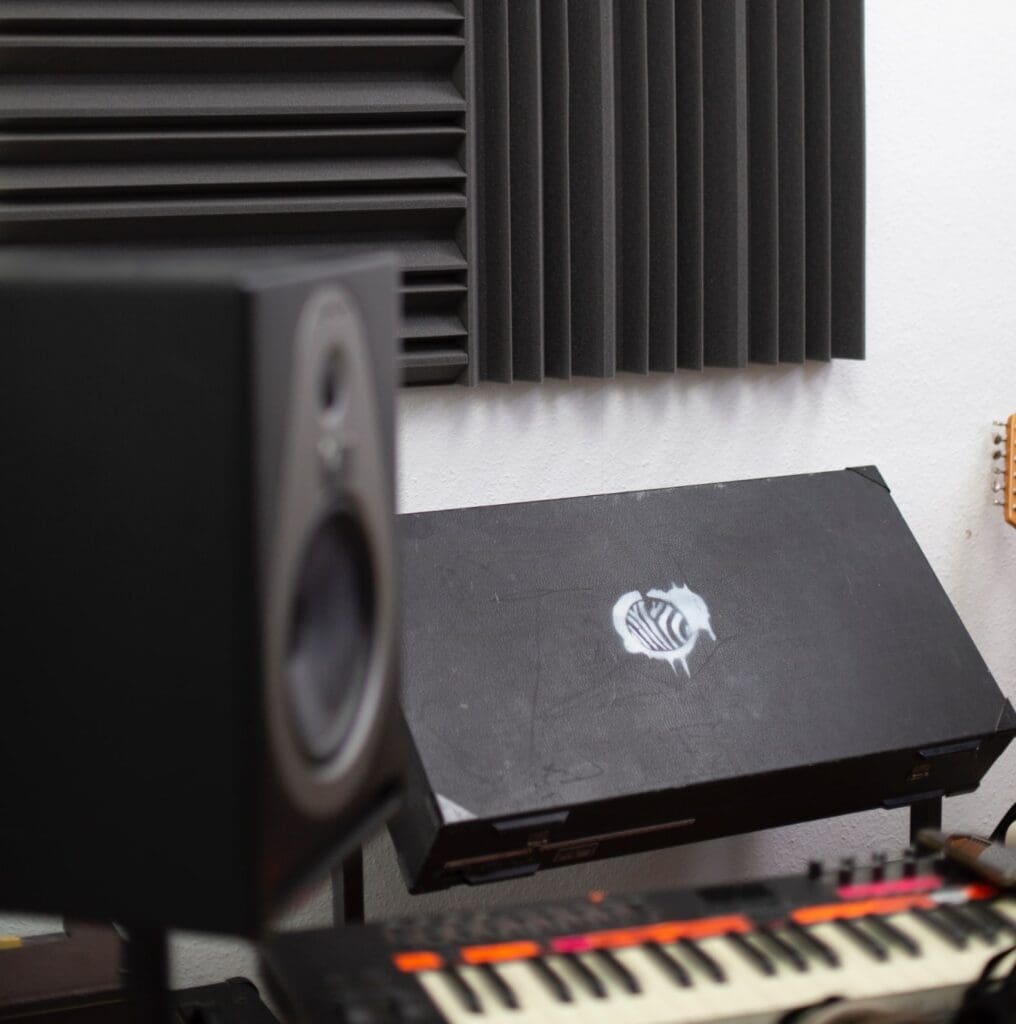
Early reflections (ER) are an acoustic problem that’s especially relevant in the control room. Because they take a “detour” via the side or rear walls, these reflections reach the ears with a slight delay. When early reflections contain a lot of energy and the distance is large enough, they are clearly perceptible as echos. Most commonly, however, they overlap with the direct sound and color it. This is also known as a comb filter effect. When it happens, even the best studio monitor speaker cannot deliver its full potential.
The acoustic treatment of a room does not typically aim to completely eliminate all sound reflections. The goal ist to guide and control them in order to avoid the aforementioned problems.


In studio control rooms, the goal is to prevent strong reflections in the listening position. Thus, they must be deflected or reduced. You can do basic acoustic planning even without performing complex calculations. Imagine that flat surfaces are mirrors and sound is light. You’ll find that angled walls and ceilings, as well as various materials, help to prevent or deflect reflections. Ideally, the listening position becomes a reflection-free zone (RFZ).
Early reflections cause the most problems in the mid and high frequency ranges. In the low frequency range, the light analogy doesn’t apply, because the wave lengths are much greater. Due to their long wave lengths, some low frequencies fit entirely or partially between two parallel surfaces in a room. This means that they lose energy more slowly and linger around for longer. The technical term for such a frequency is mode.
Modes that are spread out evenly across the frequency spectrum usually don’t have a negative effect. But when they accumulate unevenly, the room resonates at certain frequencies. At the same time, it appears like “holes” appear elsewhere in the frequency spectrum. The goal is to prevent modes from overlapping in important places in the room, such as the listening position in a control room.
In room acoustics, it is possible to specifically target certain frequencies and prevent them from building up. In other cases, bass traps are a way to achieve a broad-band attenuation in order to prevent low-frequency build-ups.
You probably know the staggered series of echoes that occur when you clap your hands in a small, empty room. That’s an example of flutter echoes. Flutter echoes are staccato-like repetitions which stand out of the diffuse reverberation and are clearly perceptible. Flutter echoes mostly occur in the mid and high frequency ranges.
When two reflective surfaces are parallel to each other, sound is reflected back and forth between them without losing energy quickly. These surfaces don’t have to be walls. Flutter echoes can also occur between the floor and the ceiling.
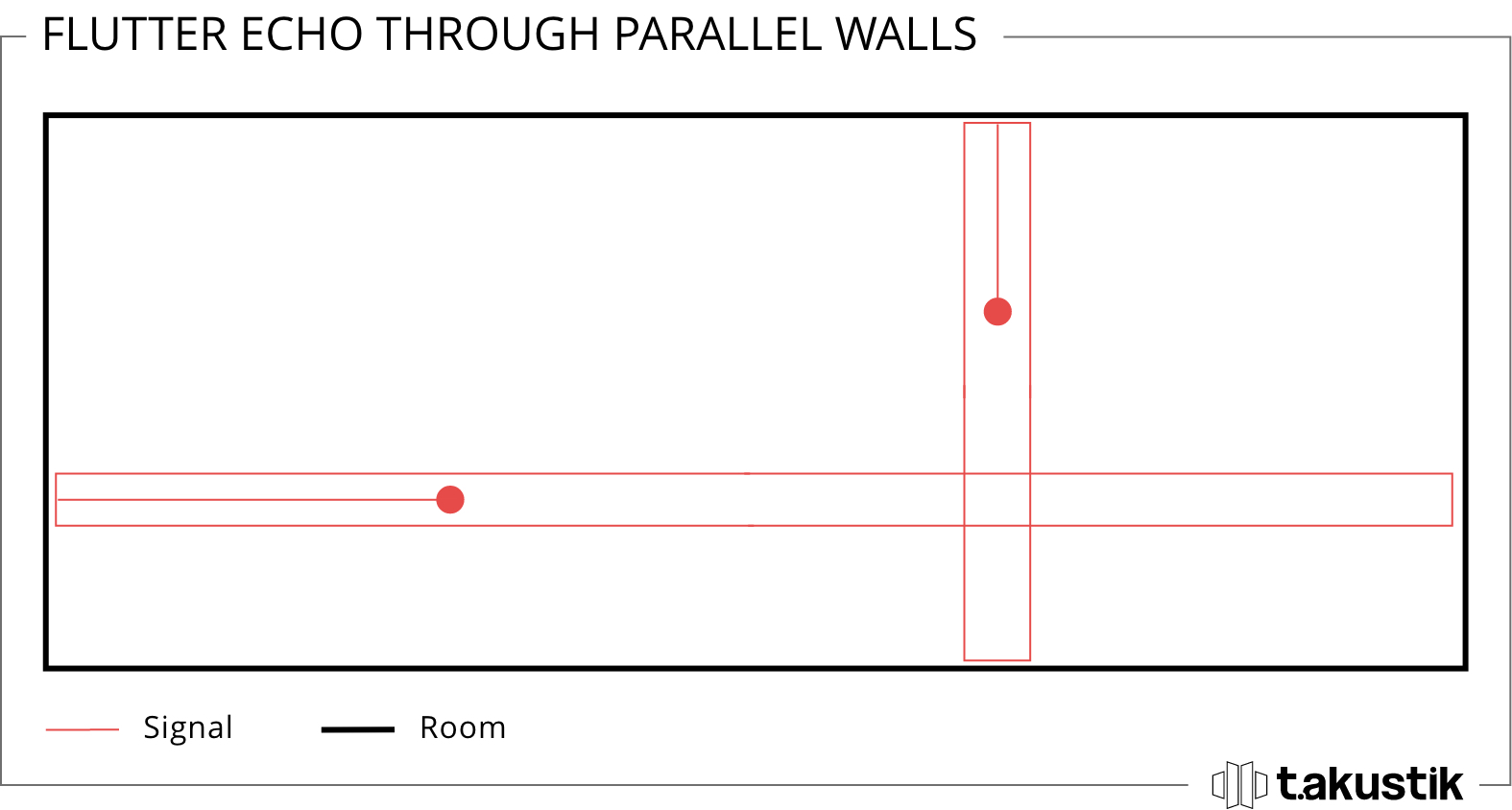
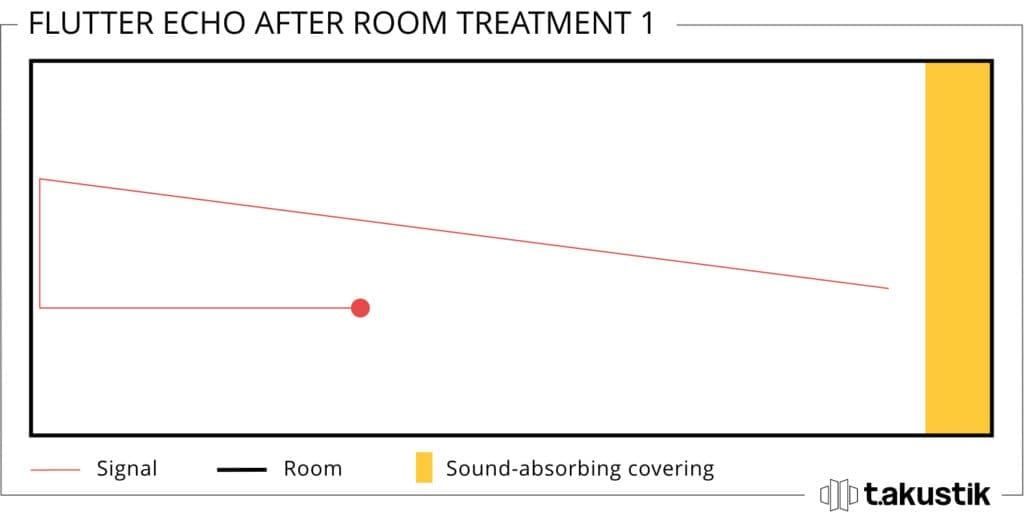
If the surfaces are close to each other, the series of repetitions is very closely spaced and can take on a tonal character. This is especially annoying, in the control room as well as during recording.
It’s almost impossible to measure flutter echoes. But it’s possible to determine the most problematic frequencies by taking the distance between the surfaces and the speed of sound into account.
In order to rule out flutter echoes from the get-go, control rooms are often designed with as few parallel surfaces as possible. This effectively prevents flutter echoes from spreading. But in a room that hasn’t been built as a control room, it can be necessary to remove unwanted flutter echoes.
While it’s possible to divert sound, this should be done on a large scale. And when you make improvements to the room acoustics, you often combine several problems. That’s why diffusion and absorptions are good options. Diffusion spreads out the sound waves that hit a surface in a diffuse pattern. Absorption “swallows” a portion of the sonic energy.
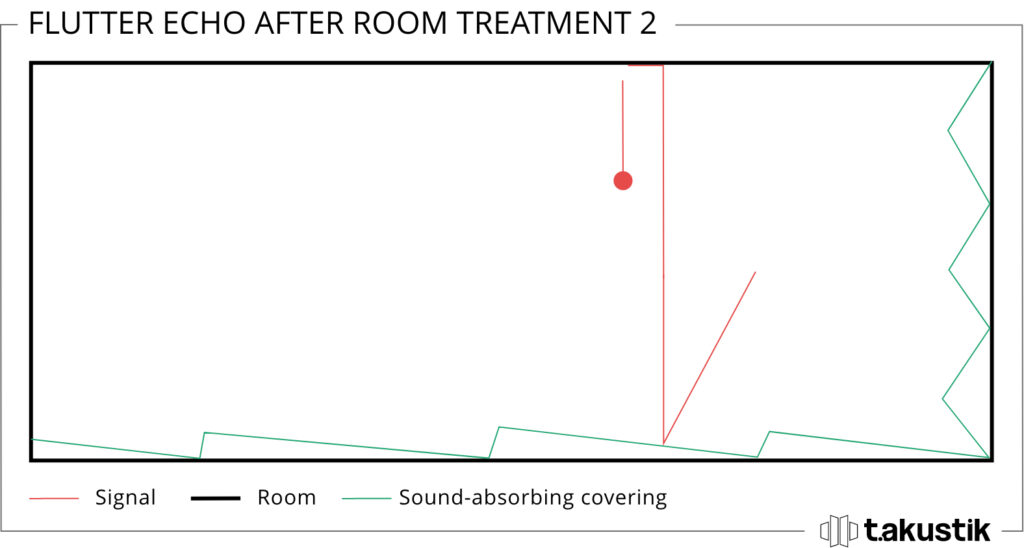
In addition to special, resonant designs, acoustic room treatment relies heavily on so-called porous absorbers. These are open-pored or open-celled structures which let air pass through, but apply some resistance. This friction converts sonic energy into heat energy and slows down the movement of the air. This is what we call absorption. Materials used include PU foams, melamine resin foams and mineral wool. They all have the same working principle, but quite different sonic characteristics.
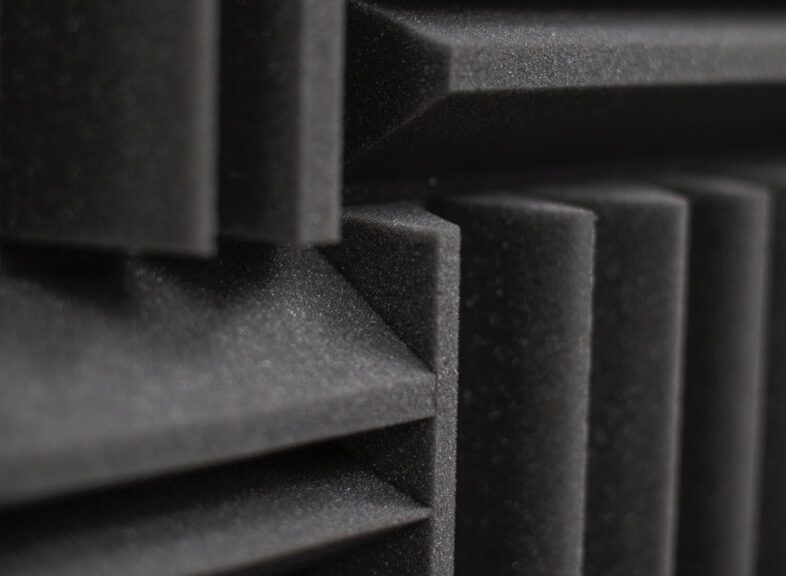
Polyurethane
Polyurethane (PU) is a frequently used synthetic material that can be foamed. Its open-pored characteristics are vital for the use in absorbers. Ether-based polyurethane is very durable. Despite its high stability, PU foam is elastic, doesn’t break and is very light.
This combination, together with the low cost, makes PU foam an efficient material for acoustic absorbers. PU foams are easy to cut, glue and mount using fasteners. While polyurethane foams are available in a variety of colors, you should not paint them yourself. This would destroy the open-pored structure.
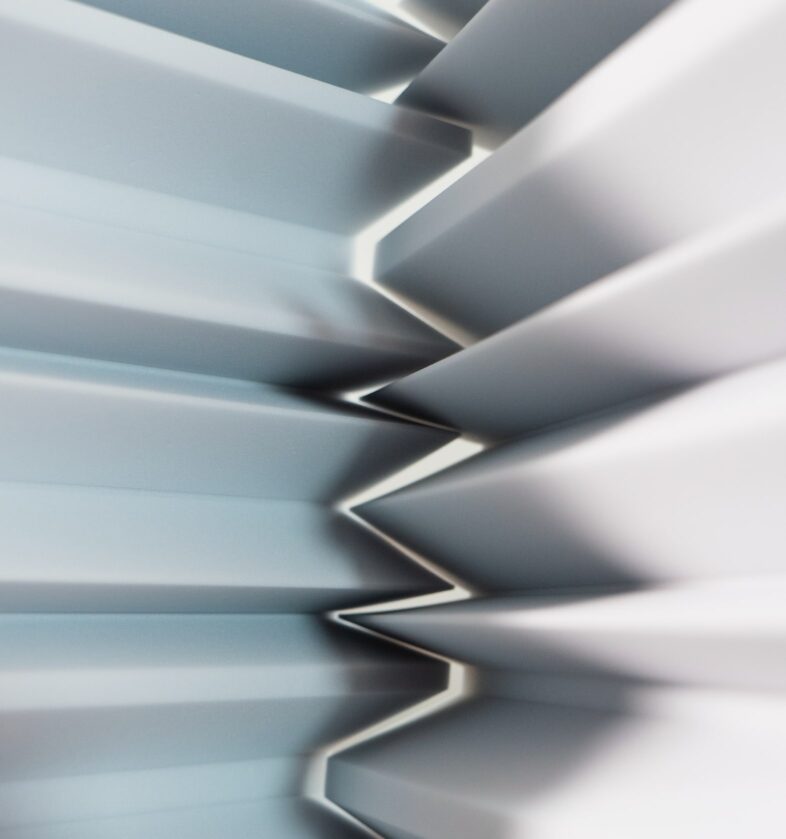
Basotect
Basotect is the brand name of an open-pored foam made by BASF SE, which consists of melamine resin and is frequently used in absorbers. Basotect is lighter than most PU foams, offers better temperature insulation and is less flammable. For these reasons, it is often used in industrial applications.
It is also less flexible than PU foam, which is both an advantage and a disadvantage. Basotect panels can be hung like a picture, stood upright in corners or used in paneled ceilings. However, they break much more easily and can crumble a bit. In contrast to PU foam, it is not possible to screw them to the wall.
Many absorbers made from Basotect panels have an adhesive backing and a membrane covering on the front side, which increases low frequency absorption and makes them more stress resistant. Basotect is also available in white, which increases the visual design options. However, it should not be painted, as that would destroy the open-pored structure.
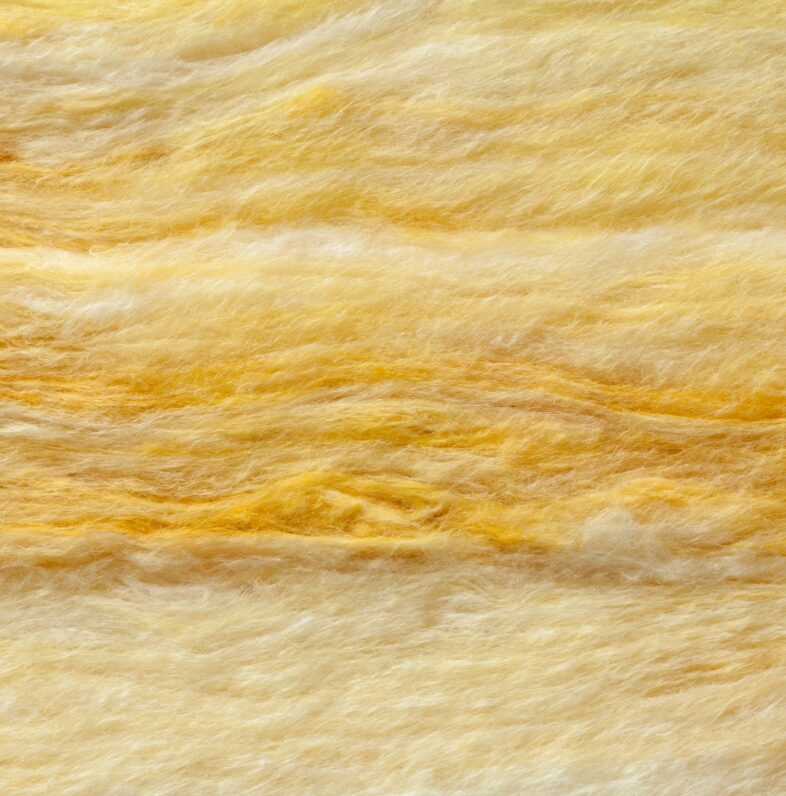
Mineral Wool
Mineral wool is a classic material for soundproofing and insulation and is widely used in construction. Synthetic mineral insulation materials can roughly be categorized in glass wool and rock wool, which use different proportions of melted rock, sand and glass.
It’s impossible to make a general assertion as to which material has the best sonic characteristics without considering the application. In acoustic applications, rock wool is often used as wall insulation, while glass wool is more often used in “open” absorbers. Both should not be used without a protective covering, as particles and dust can cause irritations of the skin and respiratory organs. This is why both are most often used in enclosed boxes and covered with cotton fabric. Since 1998, Germany has limited the use of mineral wool to varieties not suspected to be cancerous, but earlier materials may still be present in older buildings.
Some acoustic treatment materials don’t provide absorption directly through the material, but due to the construction. That’s why it is not a problem that these materials have smooth surfaces. Here are some examples of these materials:
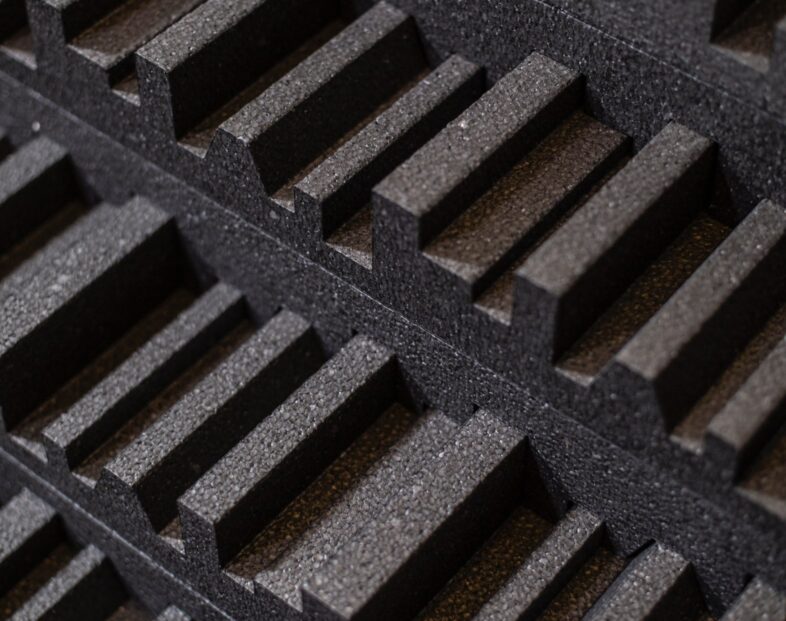
EPS
Expanded Polystyrene (EPS) is a synthetic foam best known by the brand name “Styrofoam”. In contrast to PU foam and Basotect, it is not open-pored and not suitable for use as an absorber. It is, however, used in diffusors and similar devices, which disperse the sonic energy due to their shape, while still reflecting it.
Some diffusors also have slightly absorbent characteristics. These are due to their shape rather than the material, and can usually be neglected. EPS can be painted, but care should be taken to use paint and solvents that do not harm the material.
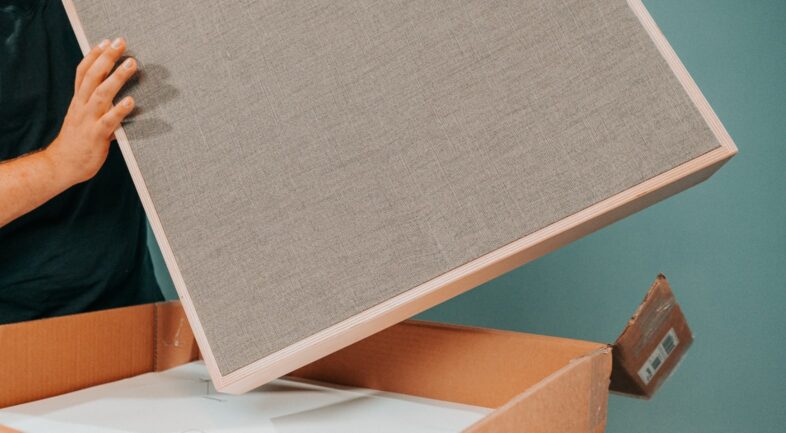
Wood
Wood is not open-pored and doesn’t absorb sound, but it is often used for framing acoustic modules. Likewise, many diffusors are made of wood, which provides an attractive and aesthetic look. Acoustic modules that use wood tend to be heavy, which is why it’s usually not possible to glue them to the wall. Wooden modules are generally bolted to the wall. Wood can be painted, varnished and stained.
Architectural trends like thermal component activation or spread-out office lofts in former factory buildings can become challenges while planning the room acoustics for office workplaces. In the case of newly constructed buildings, it’s best to consider the acoustics at an early stage during planning.
How to optimize existing offices acoustically
On the one hand, sound waves propagate on a direct path from the sound source to the listener. Therefore, blocking the direct sound is the most important step for optimizing the room acoustics in an office. This is achieved by means of tall sound screens or partitioning walls.
On the other hand, sound spreads indirectly through reflection, diffraction, and dispersion. Reflections occur on ceilings, walls, floors, and furniture. This can be minimized through the installation of appropriate absorbing materials, which “swallow” sound.
Typical measures for optimizing the room acoustics in offices:
- Sound screens between desks and corridors / other workstations (possibly ceiling-high)
- Desk-mounted absorbers
- Ceiling absorbers – as close as possible to the sound source (above the desks)
- Wall absorbers (e.g. acoustic murals) – measuring at least 1.5 m² in area, located at the reflection points on the walls
- Carpet (only effective for high frequencies)
Other acoustic measures:
- Central, acoustically isolated technical zone (copy machine, printer, etc.)
- Acoustic isolation of loud areas like conference zones and kitchenettes from the desks
- Creation of acoustically separated silent zones for undisturbed work
- Absorptive furniture
The acoustic isolating effect of partitioning walls can be improved by …
- … increasing the height of the barrier in relation to the height of the room
- … installing wider and taller sound screens
- … eliminating gaps between the screen and surrounding elements
- … moving the screen closer to the speaker or listener
- … choosing a material with a higher rate of absorption
When planning room acoustics, it must be ensured that
- the appropriate reverberation time for the room and its utilization is achieved and/or maintained
- the selected acoustic treatment options are effective in the frequency range of the human voice (125 – 300 Hz). For example: carpet typically only absorbs higher frequencies.